Toward Self-Diagnosing Bridges
Wireless, self-powered sensors may be the key to continuously monitoring infrastructure. FHWA-sponsored research is aiming to advance the technology.
Nizar Lajnef, Michigan State University
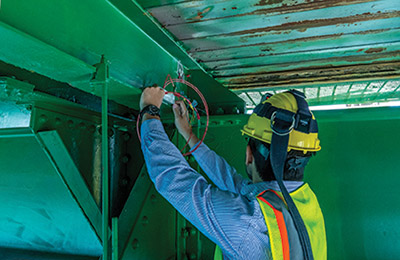
The ability to monitor the condition of bridges and other civil infrastructure systems is of primary interest to all infrastructure owners and operators. These stakeholders need to have precise and accurate information on infrastructure conditions before making decisions such as maintenance strategies or investments.
Research sponsored by the Federal Highway Administration is paving the way for wireless, self-powered sensors capable of continuously monitoring infrastructure. The development of advanced structural monitoring methods can facilitate the early detection of possible structural deterioration, with the goal to extend the lifespan of structures. Timely information gathered from such technologies will undoubtedly help transportation agencies in the corrective and urgent decisionmaking process.
Currently, state-of-the-art technologies and solutions evaluate the condition of a structure at a given interval, providing only a snapshot at the time when the measurements are taken. Results from this method are highly influenced by environmental conditions, such as ambient temperature, at the time of assessment. There are many factors that lead to bridge failures including natural hazards, crashes, overloading, and deterioration and fatigue cracking (which could result in collapse if left unchecked and could cause bridge closures). The focus of this FHWA research is to develop a unique sensor for monitoring fatigue cracks in steel bridges. These factors justify the pressing need for an autonomous, continuous, and long-term monitoring system.
One prospective solution to structural monitoring concerns is piezo-floating-gate (PFG)-based sensing technology. The sensors operate without batteries and are small enough to be embedded inside concrete or attached to steel structures. While the sensors are small, their potential to provide continuous usable data is substantial.
Challenges to Implementation
A number of challenges have hampered the practical application of structural health monitoring. Among the most serious is the infeasibility of using a sufficient number of conventional sensors (such as strain gauges and high enough accelerometers) to provide a high-enough spatial resolution to capture response variations that may be precursors to serious structural damage. The collection of measurements from the target structures needs not only the sensors themselves, but also an instrumentation network to collect and transmit the data for subsequent analysis. In addition, the need exists for robust energy sources to drive the sensors and associated data acquisition network.
The development of wireless networks has eliminated the need for performing the arduous task of stringing connecting cables. Ensuring an adequate energy source to power a sensor network for long-term, autonomous, and continuous monitoring remains a challenge. Embedded and long-term operational requirements preclude the use of batteries, whereas the small sensor volume severely limits the energy storage capacity of energy harvesting devices. Currently, a wide gap exists between the energy that can be scavenged from real-world structures and the energy density required for sensing, computing, and communication. However, this impediment has been partially resolved by research sponsored by FHWA on self-powered sensors at Michigan State University in East Lansing, MI, and Washington University in St. Louis, MO.
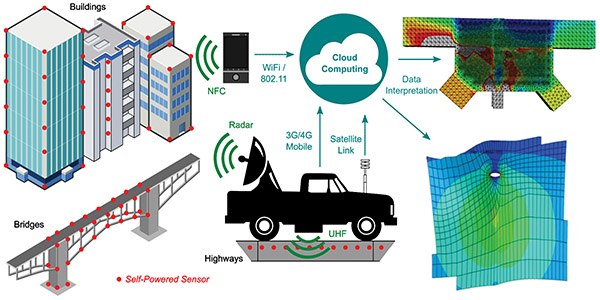
The recent research has yielded novel self-powered sensor technology that is capable of achieving picowatt power dissipation, which is two orders of magnitude lower than any other commercially available sensor technology. The ultralow power computation and data-logging technology has been shown to be capable of autonomous, long-term monitoring of dynamic loading events.
Prototype systems have been developed and tested as part of FHWA-funded projects (DTFH61-08-C-00015 Smart Pavement Monitoring System and DTFH61-13-H-00009 Ultra-low Power Wireless Sensing for Multimetric Self-Powered Monitoring of Bridge Components and Highway Infrastructure). The sensing technology offers several features that are not available in other traditional methods of structural health monitoring including: (1) low power requirements (less than 80 nanowatts of power consumption), (2) self-powered continuous sensing (no batteries required), (3) possibility of deployment in dense networks (sensor networks with a very large number of sensors to ensure no gaps in coverage) given the small size of the sensors and the fact that they do not rely on batteries, (4) autonomous computation and nonvolatile storage of sensing variables, and (5) wireless communication.
Nizar Lajnef, Michigan State University
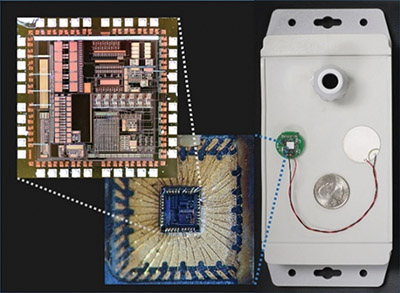
“There is huge potential and benefit for sensors like these on structures beyond the Mackinac Bridge, and we’re excited these prototypes are being tested here,” says Executive Secretary Bob Sweeney with the Mackinac Bridge Authority in Michigan. “We meticulously maintain and inspect the bridge each year, and sensors like these will complement our efforts, giving us even more information on the bridge’s condition to help keep it well-maintained and safe for many years to come.”
What Makes this Technology Unique?
All sensor technologies developed to date are based on a similar set of components, or building blocks. Typically, a system consists of a transducer to generate the signal of interest, converters and regulators, and a data storage or transfer link. These components require a constant source of power. As a result, all commercially viable sensors require either solar or battery power. Neither option is practical for monitoring highway infrastructure because it requires thousands of sensors to properly monitor the desired responses.
For pavements, sensors need to be embedded beneath the material to be able to detect hidden cracks, thus eliminating the possibility of using batteries or solar power. For bridges, a large number of sensors is needed for an effective high-density monitoring network. However, periodic replacement of batteries is unfeasible, and the expense of solar power technology is cost-prohibitive. Furthermore, in the context of civil structures systems, it is important to be able to capture each loading event. Structures are infrequently subjected to significant loading. During the times between significant loading cycles, it is possible that the sensor would experience periods of inactivity. It is a challenge to handle these “blackout” periods because commercially available solutions using any onboard battery or ultracapacitor might lose their charge between intermittent loading cycles. The same logic can explain the need for using nonvolatile memory storage because any volatile memory storage would be lost during power blackouts.
Mackinac Bridge Authority
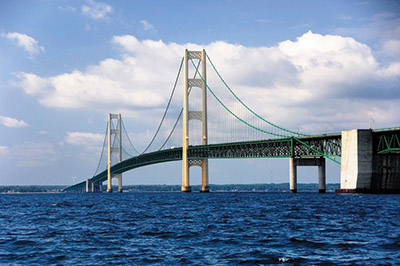
To overcome these challenges, FHWA-sponsored research has led to the invention of a new way of capturing, computing, and storing the strain and acceleration signals generated on a civil or mechanical structure. The technology is called piezo-floating-gate, or PFG, sensing. “Piezo-” is borrowed from the piezoelectric transducers used in the technology. These materials are capable of converting mechanical energy, such as vibrations, into electrical energy. The generated electrical signals, which are directly proportional to the mechanical excitation, are also used to power all electronics in the sensing system.
Mackinac Bridge Authority
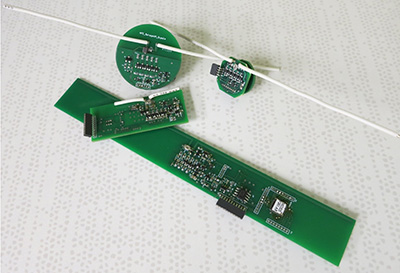
Nizar Lajnef, Michigan State University
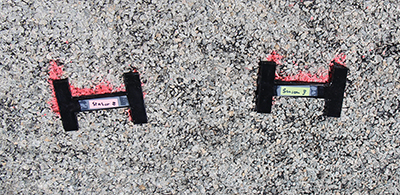
The novel design enables the sensor to derive its power from the same signal it is sensing. The sensor electronics act like a large container of electrons. Every time the structure is loaded, for example when a truck passes over a bridge or over pavement, the piezoelectric material will send a signal that will shake the container. Because of that energy, some electrons will get thrown away into a smaller container that is isolated and does not get shaken (hence the name “floating”). The process is called hot-electron injection. The smaller container acts like permanent memory because the electrons in it are permanently trapped. Counting the number of trapped electrons will convey the number of times the system was shaken and the intensity of those trembles, which is directly related to the number and amplitude of the external loading (for example, a passing truck). This concept implements a power-efficient sensing system, and alleviates the need for a power source and all other interface electronics required in a typical sensor.
The Data Challenge
Another important challenge in monitoring the health of a large structure is to make sense out of the large amount of data generated by the sensors. To address this challenge, each sensor has a series of memorycells, or gates, that are placed adjacent to the computational circuits. The information delivered by the piezoelectric transducer is stored in these gates. The gates record the duration of strain/voltage events when the amplitudes of the input signals, generated by the piezoelectric transducer, exceed certain predetermined thresholds. The output of the sensor is presented in the form of a histogram, in which each gate denotes the cumulative time of the events at a specific, predetermined strain level. The self-sustained sensing systems use the sensor output to relate the variation rate of strain distributions to the rate of damage.
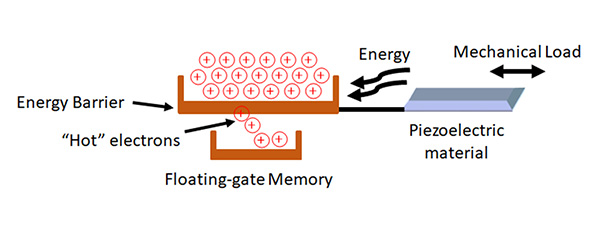
The new type of data requires specific techniques for data interpretation. The research team at Michigan State University has developed advanced software and tools for bridge operators and State departments of transportation to understand the data. The software will automatically interpret the data and then synthesize and present them in a simplified way to users to assist them when making maintenance decisions.
The successful development and implementation of this multimetric sensing system and the data interpretation procedures could dramatically transform the economics of bridge and road preservation, and ultimately improve infrastructure serviceability.
Fred Faridazar is a highway research engineer with the FHWA Office of Infrastructure Research and Development at the Turner-Fairbank Highway Research Center, a position he has held since 2000. He has acquired research experience related to structures, pavements, concrete materials to complement the bridge design and construction experience acquired through his prior positions with the FHWA Florida Division Office and FHWA’s Office of Federal Lands Highway.
For more information, see https://highways.dot.gov/research/turner-fairbank-highway-research-center/facility-overview or contact Fred Faridazar at 202–493–3076 or Fred.Faridazar@dot.gov.