Texas High-Strength Concrete Bridge Project
Introduction
High-strength concrete is one of the most significant new materials available to federal, state, and local highway agencies to rehabilitate the nation's crumbling infrastructure. High-strength concrete, as defined in this article, has a specified design strength of 55.2 megapascals (8,000 pounds per square inch) or greater. With its improved impermeability, durability, and accelerated strength gain over normal-strength concrete, high-strength concrete should enhance performance and be an ideal material to assist with the widespread problem of deteriorating bridge structures.
In addition to its better long-term performance, the use of high-strength concrete allows either longer spans with fewer support locations or fewer beams for a given span length. This results in savings due to reduced fabrication, transportation, and erection costs, as well as reduced substructure requirements because of the lighter superstructure. The net result should be comparable, if not decreased, costs relative to conventional construction.
This article describes the design and construction details for Louetta Road Overpass, two adjacent bridges on State Highway 249 in Houston, Texas. These structures showcase the use of high-strength concrete and are the first bridges in the United States to fully use high-strength concrete in all aspects of design and construction. Several innovations make these structures unique in the use of high-strength concrete. They are also the first in the United States to use 15.24-millimeter- (0.6-inch-) diameter, prestressed strands in a pretensioned concrete application and on a 50-mm (1.97-in) grid spacing.
A number of differences exist between the Louetta bridges and the Braker Lane Bridge, built in Austin, Texas, in 1990. The Braker Lane Bridge is a conventional prestressed concrete beam bridge except that its I-shaped beams have a design strength of 66.2 MPa (9600 lbf/in2). Actual concrete strengths ranged from 75.8 MPa (11,000 lbf/in2) to 96.5 MPa (14,000 lbf/in2) at 28 days.
The Louetta Road Overpass structures, which were let to contract in February 1994, are simple-span, pretensioned concrete beams with composite precast/cast-in-place concrete decks using construction materials that incorporate the latest technology. All components of these structures are being built with high-strength concrete. The beams are pretensioned concrete U-beams -- a recent, Texas Department of Transportation (TxDOT) development in which aesthetics was combined with the usual safety, economy, and durability concerns to form the primary driving forces for design. Rather than using typical prestressed concrete design strengths in the traditional 34.5- to 41.4-MPa (5,000- to 6,000-lbf/in2) range, the beams in these structures fully use concrete strengths in the 69- to 89.6-MPa (10,000- to 13,000-lbf/in2) range.
The high-strength beams combine with a high-strength concrete deck in one of the structures to obtain a totally high-strength concrete superstructure. The superstructures are supported on high-strength concrete, post-tensioned pier segments -- another new application for conventional bridge construction. This culminates in the first bridge structures in the United States to fully use high-strength concrete in combination with aesthetics and economy in a total package that adds up to long-term high performance at costs that are anticipated to be competitive with conventional structures. It is expected that information developed from this project will assist in the design of high-performance, economical structures throughout the country in the near future and for years to come.
Other High-Strength Concrete Bridges*
1980 |
Red River Cable-Stayed Bridge Guangxi, China |
60 MPa (8,700 lbf/in2) |
---|---|---|
1985 |
East Huntington Cable-Stayed Bridge East Huntington, W.Va. |
55 MPa (8,000 lbf/in2) |
1986 |
Annacis Cable-Stayed Bridge Vancouver, British Columbia, Canada |
55 MPa (8,000 lbf/in2) |
1989 |
Joigny Bridge Joigny, France |
60 MPa (8,700 lbf/in2) |
1990 |
Braker Lane Bridge Austin, Texas |
66 MPa (9,600 lbf/in2) |
1990 |
Liangshui River Bridge Beijing-Tianjin Highway, China |
60 MPa (8,700 lbf/in2) |
1993 |
Normandie Bridge Normandie, France |
60 MPa (8,700 lbf/in2) |
1993 |
Portneuf Bridge Quebec, Canada |
60 MPa (8,700 lbf/in2) |
The Cooperative Agreement
In July 1993, a cooperative agreement was initiated between the FHWA and TxDOT, in conjunction with the Center for Transportation Research (CTR) at The University of Texas at Austin, to conduct research in the design and construction of extra-high-strength concrete bridges. The agreement involves the Louetta Road Overpass with specified design strengths in the 69- to 89.6-MPa (10,000- to 13,000-lbf/in2) range for pretensioned beams and 69 MPa (10,000 lbf/in2) for post-tensioned substructures. This research project is the first phase of a larger, overall effort to design and construct bridges with specified strengths in the 103.4- to 117.2-MPa (15,000- to 17,000-lbf/in2) range. The emphasis is to collect data on all aspects of high-strength concrete bridge construction to lay the groundwork for designing and constructing extra-high-strength concrete bridges with minimum variation from conventional bridge-building techniques. A cooperative agreement is also anticipated between FHWA and TxDOT to develop and present technology transfer materials in conjunction with the Louetta Road Overpass design and construction effort.
Louetta Structures
The existing S.H. 249 is a four-lane, at-grade, asphalt-surfaced road that is classified as a major rural/urban arterial. After reconstruction, the thoroughfare, known as the Aggie Freeway, will be a controlled-access, six- to eight-lane, divided freeway with access ramps to and from frontage roads and with a number of mainlane overpasses, including Louetta Road. The 1992 average daily traffic count for S.H. 249 was 32,700 vehicles, of which 5.8 percent -- approximately 1900 vehicles -- were trucks. A minimum average daily traffic count of 144,200 vehicles is projected for the year 2022, again with 5.8 percent -- 8400 vehicles -- projected to be trucks. The construction phasing allows the project to proceed with minimum inconvenience to motorists.
The sequence of work was modified to allow construction of the Louetta Road Overpass structures at the beginning of this project. Construction is, therefore, anticipated to start in the spring of 1994 and to be completed in the fall of 1994. According to the current plan, the structures will be opened to traffic in 1995.
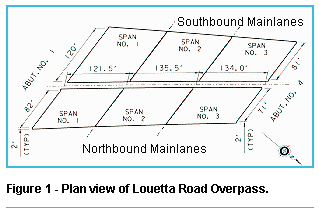
Figure 1 shows a plan view of the Louetta structures. Each three-span unit consists of a 37-m (121.5-ft) span, a 41.3-m (135.5-ft) span, and a 40.8-m (134-ft) span, each with a varying roadway width. These lengths are longer than the maximum spans used in typical U-beam bridges, which have wide beam spacings that limit the span lengths to approximately 35.1-m (115-ft).
Thus, the Louetta bridges are an ideal application for high-strength concrete. The longer span lengths combine with wider spacing of the U beams to provide a more structurally efficient, aesthetic, streamlined appearance than could be achieved with typical design concrete strengths of less than 55.2 MPa (8,000 lbf/in2). Six U-beams span the southbound lanes, and five U-beams span the more narrow northbound lanes. The average beam spacings vary from 3.57 to 4.82 m (11.7 to 15.8 ft), as shown in table 1. This results in clear spacings of 1.13 to 2.38 m (3.7 to 7.8 ft) between beams.
Table 1 - Average beam Spacings and Concrete Strengths
Category |
Unit |
Southbound |
Northbound |
---|---|---|---|
Avg. Beam Spacing ~ Span #1 |
ft | 15.8 | 13.0 |
Avg. Beam Spacing ~ Span #2 |
ft | 13.7 | 12.4 |
Avg. Beam Spacing ~ Span #3 |
ft | 11.7 | 11.7 |
f'c ~ U-Beams [3 designs] |
psi | 6,900 to 8,800 | 6,900 to 8,800 |
f'c ~ U-Beams [3 designs] |
psi | 9,800 to 13,100 | 9,800 to 13,100 |
f'c ~ Post-Tensioned Piers |
psi | 10,000 | 10,000 |
1 ft = 0.3048 m
1 psi (lbf/in2 = 6.89 kPa
Also shown in table 1 are the specified design concrete strengths. The U-beams are designed for three concrete mixes, with design release strengths from 47.6 to 60.7 MPa (6,900 to 8,800 lbf/in2) and with specified 56-day design strengths from 67.6 to 90.3 MPa (9,800 to 13,100 lbf/in2). The post-tensioned concrete pier segments are designed for 69-MPa (10,000-lbf/in2), specified 28-day strength. The design strengths for the U-beams were specified at 56 days, the project standard for high-strength concrete. The standard is 56 days, compared to the 28-day standard for normal-strength concrete, to account for the appreciable strength gain with time that occurs in high-strength concrete after 28 days. The post-tensioned concrete segments, however, were specified at 28 days because preliminary results from the 69-MPa (10,000 lbf/in2) mix designs indicated the strength could be achieved in 28 days.
The U-Beam
The Louetta structures were designed using a new beam shape recently developed by TxDOT. Dubbed the "U-beam", this beam is a pretensioned concrete, open-top, trapezoidal-shaped beam that was developed as an economical, aesthetic alternative to I-shaped beams. Structures designed with U-beams typically require only one-half to two-thirds as many beams as structures designed with I-beams.
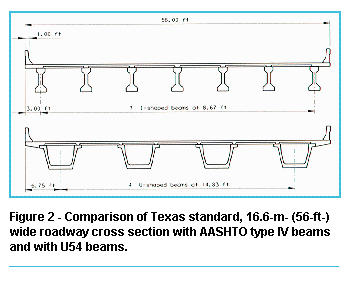
A reduction in both number of beams per span and number of visual horizontal breaklines per beam contributes to the streamlined appearance of the U-beam bridge. This can be seen in figure 2, which shows that seven American Association of State Highway and Transportation Officials (AASHTO) Type IV beams -- 1.37-m- (54-in-) deep prestressed concrete I-beams -- on a typical 17.1-m- (56-ft-) wide roadway can be replaced with four 1.37-m (54-in) U-beams, called U54 beams. The Louetta bridges, designed with high-strength concrete U54 beams, will have a similar decrease in the number of beams.
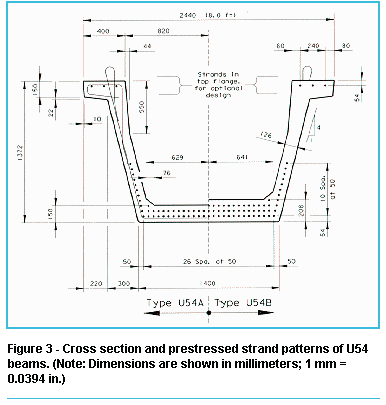
The U-beam was developed in metric dimensions, anticipating the requirement that all federally funded construction projects be specified in metric (SI) units, effective September 1996. Figure 3 shows the cross- sectional dimensions and pretensioned strand locations for the U54 beam. This beam is 1372 mm (54 in) in depth, thus allowing its use as an alternative to the 1372-mm (54-in) AASHTO Type IV beam, the most common I-beam used in Texas bridge construction. The 2440-mm (8.01-ft) overall top flange width includes two 400-mm- (15.75-in-) wide compression flanges. Two or three layers of prestressed strands, with a maximum of 27 strands per row, can fit in the 1400-mm- (5-ft-) wide bottom flange, as shown in figure 3. A single strand per row may be placed in each of the 126-mm- (5-in-) wide webs.
High-Strength Concrete
The economical use of high-strength concrete depends on a local supply of acceptable aggregate, as well as on other considerations, such as quality of available cement, admixture compatibility, and availability of mineral admixtures. Because this structure is in a no-freeze area, it was not necessary to use air entrainment, which normally carries a sacrifice in strength.
The Economical Use Of High-Strength Concrete Depends On A Local Supply Of Acceptable Aggregate.
One of the most important considerations for this project was the understanding of the role of the aggregate on the performance of the concrete. Optimization of the physical characteristics of the aggregate resulted in lower mixing-water demand while retaining adequate workability, higher strengths, higher modulus of elasticity, and higher flexural strengths. For the same materials and mix proportions, the aggregate resulted in up to 24.1-MPa (3,500-lbf/in2) strength difference and up to 20-percent difference in modulus of elasticity. Of great importance to optimizing the flexural strength of the concrete were the surface characteristics, size, and composition of the aggregate, as well as the amount used in the concrete.
The strength-gain characteristics of the concrete were greatly influenced by the type and dosage rate of retarding admixture, as well as by the compatibility between the retarding admixture and the high-range water-reducing admixture. Critical to the performance of the concrete was also the time of addition of each admixture, as well as the minimum allowable mixing-water content of the concrete. Cement content was found to be ineffective above about 294.84 to 317.52 kilograms (650 to 700 pounds) of cement per 0.765 cubic meter (one cubic yard). Instead, the use of a high-quality ASTM Class C fly ash was found to be necessary to obtain adequate workability, placeability, and concrete strength at early ages. Fly ash contents of up to 40- percent replacement of the cement were evaluated.
Use of 15.24-mm- (0.6-in-) Diameter Strand
To take full advantage of high-strength concrete, a higher prestress force is required than can typically be achieved with the use of 12.7-mm- (0.5-in-) diameter prestressing strand. Therefore, the Louetta Road Overpass beams have 15.24-mm- (0.6-in-) diameter prestressed strands. Also, the strands are on the same 50-mm (1.97-in) grid spacing as used for standard 12.7-mm- (0.5-in-) diameter strands to maximize the effect of the larger strand and to allow the use of existing hardware at the precast plants. This grid spacing results in a clear spacing between strands that is less than currently allowed in AASHTO standard specifications. Although FHWA has a moratorium on the use of 15.24-mm- (0.6-in-) diameter strands for pretensioned applications a special approval was requested and received from FHWA based on results from tests conducted on this project indicating no problems with the strand configuration.
A 42-percent increase in prestress results from using the 15.24-mm- (0.6-in-) diameter strand. This increase in prestress force allowed full use of concrete strengths greater than 69 MPa (10,000 lbf/in2) in the U-beam designs. The benefit of this combination quickly became apparent in designing the U-beams for the Louetta structures. The interior beams in the 37-m (121.5-ft) span of the northbound mainlanes were the only beams that could be designed with 12.7-mm- (0.5-in-) diameter prestressed strands. All other beams required 15.24-mm- (0.6-in-) diameter strands in combination with the high-strength concrete to meet design criteria.
Use of the larger 195.5 kN (43,950 pounds force) per strand on a 50-mm (1.97-in) grid spacing raised concerns about stress concentrations and possible concrete splitting in the end regions of the beams and about development length requirements. To get field data and to verify adequate performance, two full-scale U-beam specimens were cast in September 1993. The beams were instrumented with mechanical gage points on the outside surfaces of the webs along the beam length to measure concrete strains at release of prestress. No end splitting due to prestress force was seen, and preliminary results from strain measurements indicate adequate transfer length. The release was gradual (multistrand release), as required by TxDOT for bridge projects.
Also of concern is the development length of 15.24-mm- (0.6-in-) diameter strand. Two rectangular beams, 355.6 mm (14 in) wide and 1,066.8 mm (42 in) deep, were cast in December 1993. Each was pretensioned with one layer of six 15.24-mm- (0.6-in-) diameter strands on a 50.8-mm (2-in) grid spacing at 50.8 mm (2 in) up from the bottom fiber. These beams will be tested to failure at the CTR laboratory to obtain development-length measurements.
Beam Design
The beam designs have different concrete design parameters due to the use of high-strength concrete. The allowable tension coefficients were increased from 7.5 to 10 for release and from 6 to 8 for final to be consistent with previous experimental studies of high-strength concrete. Modulus of rupture tests conducted on the mix designs used in the field tests indicate an allowable tension coefficient at release of 9.6.
The modulus of elasticity used in design was 41,370 MPa (6 million lbf/in2) at seven days. An average modulus of elasticity of 45,507 MPa (6.6 million lbf/in2) at seven days was obtained in the field tests.
Concrete Mix Proportioning
When proportioning high-strength concrete, emphasis must be placed on the interaction among the components used in making the concrete, as well as the quality and characteristics of each component. Materials selection should be based on the following four considerations:
- Contribution to the workability, placeability, and finishability of the fresh concrete.
- Effect on the mixing-water demand of the fresh concrete for adequate workability.
- Effect on the mortar-aggregate tensile bond strength within the hardened concrete.
- Contribution of the material to any special specification or performance requirements of the concrete, such as durability, modulus of elasticity, or flexural strength.
The special requirements for the Louetta U-beams include a high modulus of elasticity, 41,370-MPa (6-million lbf/in2) minimum at early ages to control deflections, as well as a 33-percent higher flexural strength, as indicated using the modulus of rupture test. This increased demand on the flexural capacity of the concrete was needed to prevent cracking at release. Other specifications include concrete compressive strengths up to 60.7 MPa (8,800 lbf/in2) at release, which is typically 18 to 24 hours after casting and up to 90.3 MPa (13,100 lbf/in2) at 56 days.
The concrete is also required to have excellent workability to allow placement from one web of the U-beam. The concrete must flow across a 1400-mm- (4.59-ft-) wide bottom flange without segregation, passing two layers of strands in a 158-mm (6.22-in) depth or three layers of strands in a 208-mm (8.19-in) depth, and then flow into the bottom portion of the far web, at which time concrete is placed in the far web to fill the entire cross section.
No silica fume was used in any of the mixes in this study.
A field trial batch program was conducted to evaluate eight of the mixes that had been developed in the laboratory. The results from the trial batch program are detailed below. No accelerated curing was used in the field tests.
In summary, use of high-strength concrete depends greatly on the optimization and engineering of the concrete and its components. For high-strength concrete construction, compressive strength, flexural strength, modulus of elasticity, creep, and shrinkage need to be predictable performance characteristics of the concrete. It is evident that future advancements in construction are going to be highly dependent on advancements in concrete technology.
FRESH CONCRETE | |
Slump after high-range water |
177.8 to 241.3 mm (7.0 to 9.5 in) |
---|---|
Concrete temperature: |
35.6° to 38.9° C (96° to 102° F) |
Unit weight: |
66.77 to 69.67 kg/m³ (147.2 to 153.6 lb/ft³ [pcf]) |
HARDENED CONCRETE | |
Compressive strength |
56.677 to 66.054 MPa (8,220 to 9,580 lbf/in²) |
Compressive strength |
90.118 to 110.32 MPa (13,070 to 16,000 lbf/in²) |
Modulus of elasticity |
41,370 to 48,270 MPa (6 to 7 million lbf/in²) |
Pier Design
The Louetta substructures at interior bent locations are designed as individual post-tensioned piers, as shown in figure 4. The hollow core column segments are 0.99 m (3.25 ft) square and have 228.6-mm (9-in) daps at the corners. The two transverse walls are 101.6 mm (4 in) thick. The two longitudinal walls are 190.5 mm (7.5 in) thick, with each containing three 34.93-mm- (1.375-in-) diameter post-tensioned bars. The post-tensioned bars are designed for a wobble coefficient of 0.0, an anchorage set of 1.59 mm (0.0625 in), a maximum average bar stress after anchoring of 70-percent guaranteed ultimate tensile strength, and allowable final concrete stress coefficients of 3 for tension and 0.4 for compression.
This innovative substructure was selected for several reasons. First, the bridges are classified as highly visible, and therefore, an aesthetic substructure as well as superstructure was required. The individual piers, devoid of the conventional connecting cap, are perceived by many as more aesthetic. Second, relatively thin-walled, hollow post-tensioned concrete pier segments were required to fully use the high concrete strength in design and to also have a practical and economical construction method. In addition, the use of precast hollow pier segments allows the introduction of architectural creativity in future designs. Third, the time savings inherent with precast concrete construction should result in a reduction in costs. Immediately upon completion of the pier construction, the U-beams may be erected and deck construction begun. This saves a significant amount of construction time since the substructure does not require the usual seven-day minimum to obtain design concrete strengths.
Deck Design
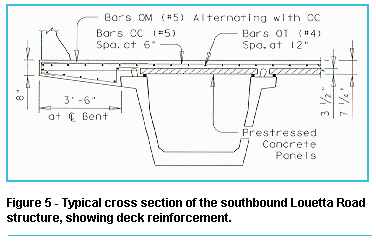
Figure 5 shows a typical partial transverse section of the southbound mainlanes of the Louetta Road Overpass. The cast-in-place reinforced concrete in the deck was specified as 55.16-MPa (8,000-lbf/in2) concrete on the southbound mainlanes and as the Texas standard 27.6-MPa (4,000-lbf/in2) concrete on the northbound mainlanes. Both structures have 55.2-MPa (8,000-lbf/in2) prestressed concrete panels specified, although permanent metal deck forms are allowed as an option. The 55.2-MPa (8,000-lbf/in2) concrete on the southbound mainlanes was specified so that observations could be made to determine whether the increased cost and complexities of placing and curing the higher strength concrete are justified by an increase in long-term performance.
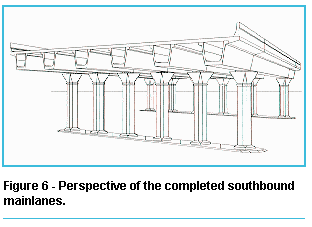
The deck was designed for main reinforcement perpendicular to traffic, the standard method used in Texas. The transverse No. 5 bars at 152.4-mm (6-in) spacings were doubled on the overhang. This allowed a 2.29-m (7.5-ft) overhang from centerline of outside U-beam to edge of slab, compared to the 2.06-m (6.75-ft) overhang used in standard U-beam bridge design. The wider overhangs contribute to a more aesthetic overall appearance.
A perspective of the completed southbound mainlanes is shown in figure 6, and an artistic rendering of both structures is shown in figure 7.
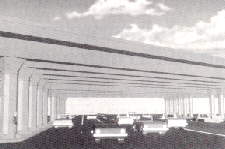
Quality Control/Quality Assurance Program
Most important to the successful completion of a high-strength concrete project is the establishment of a comprehensive Quality Control/Quality Assurance (QC/QA) program. Furthermore, if the QC/QA program is to work as intended, it must be developed with the full cooperation and approval of all the parties involved in the construction process including FHWA, the state DOT, and the general contractor and its subcontractors, such as the testing laboratory and the concrete supplier.
Developing and implementing the QC/QA program will be a dynamic process requiring continuous updating through regularly scheduled meetings to accommodate test results and findings during actual construction. The QC/QA program should cover all aspects of the concreting process at the construction site from materials selection to concrete production, curing, testing, placement, troubleshooting, and performance evaluation. This program should include some of the more traditional aspects of quality control, such as frequency of testing, sampling, size of test specimens, testing machines, sample preparation, and others as well as strict, fresh concrete temperature controls, limits on temperature rise due to cementitious hydration, vibration, surface temperature at time of formwork removal, etc.
Key elements to the QC/QA program are the awareness of the parties involved of the purpose of each of the elements of the QC/QA program and their commitment to communicate and cooperate in the resolution of any concerns. The Louetta project will be an opportunity for all individual parties to contribute to the overall QC/QA plan and ensure the success of this challenging and unique bridge project.
Technology Transfer Efforts
Efforts are underway to document all aspects of the Louetta Road Overpass high-strength concrete project. Videotapes and slides have been taken of the two castings of research beams done at the precast plant and are planned for the preconstruction and subsequent meetings, the U-beam and pier segment fabrication, the bridge construction, and any loading or monitoring of the completed structures.
The videotapes with script will be edited into a 10-15 minute production that documents the entire project. The slides are being used in presentations at various technical conferences and meetings around the country.
Ongoing efforts include development of brochures on the initial and final phases of this high-strength concrete project and development of two or three multiday workshops on high-strength concrete to be held around the country, including one planned for Houston during bridge construction.
Future Research
Future FHWA-sponsored, concrete-related research will focus on developing:
- High strength concrete.
- Durable frost-resistant concrete for cold environments.
- Higher-load-carrying capacity strands to reduce steel congestion.
- Evolving technical details for handling and connecting these higher capacity members.
Conclusion
The Louetta Road Overpass structures are the first in the United States to fully use high-strength concrete in all aspects of design and construction. They are also the first in the United States to use 15.24-mm- (0.6-in-) diameter strands in a pretensioned concrete application and on a 50-mm (1.97-in) grid spacing. This project lays the groundwork for designing and constructing extra-high-strength concrete bridges with minimum variation from conventional bridge-building techniques.
The benefits of high-strength concrete to the transportation system are being identified, documented, and verified in this project. Methods for optimizing all aspects of design and construction are being developed, and specifications and construction documents for future high-strength concrete projects are being formulated. Results from this project should assist the engineering and construction professions in consistently producing high-strength, high-performance, prestressed concrete bridges and to do so at competitive costs.
Mary Lou Ralls, P.E., is a supervising design engineer in the Bridge Section of the Design Division of the Texas Department of Transportation. She is technical chair of the Louetta research project and has been coordinator for the development of the U-beam. She received a bachelor of science degree in civil engineering and a master of science degree in engineering from The University of Texas at Austin, and she is a registered professional engineer in the state of Texas.
Ramon Carrasquillo, Ph.D., P.E., is a professor of civil engineering at The University of Texas at Austin. He also serves as the associate director of the Center for Aggregate Research and coordinator of the Construction Materials Graduate Program. He is very active in professional organizations such as the American Concrete Institute and the American Society for Testing and Materials. Since 1976, high-strength concrete has been a major topic of his research program.