The Case for Cutting-Edge Construction in Puerto Rico
PRHTA is committed to turning geosynthetic reinforced soil integrated bridge systems from innovation to standard practice. Here’s a look at its first project.
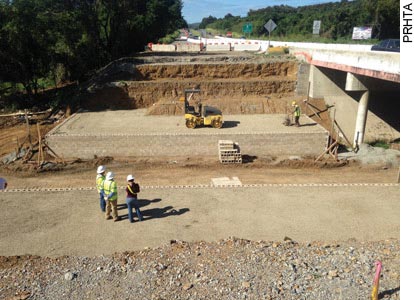
Much like the rest of the country, Puerto Rico faces an inventory of deteriorating bridges and a limited budget for bringing them up to par — not to mention building new ones. To help stretch each dollar further, in December 2010, representatives from the Puerto Rico Highway and Transportation Authority (PRHTA) joined other stakeholders at the Every Day Counts (EDC) regional summit in Atlanta, GA. While there, the PRHTA participants selected a number of innovations to help them do more with less.
To encourage implementation of EDC initiatives, the Federal Highway Administration (FHWA) partnered with the American Association of State Highway and Transportation Officials (AASHTO) to host 10 regional summits. Partners and stakeholders that supported the 1.5-day summits included the Associated General Contractors of America, the American Road & Transportation Builders Association, the National Association of County Engineers, and the American Public Works Association.
Among the innovative technologies that PRHTA selected during the summit was the implementation of geosynthetic reinforced soil integrated bridge systems (GRS–IBS), which use alternating layers of compacted granular fill material and geosynthetic reinforcement. The agency also committed to implementing several other technologies, such as warm-mix asphalt and accelerated bridge construction (ABC) using prefabricated elements.
“After learning of the EDC initiative, our engineers were extremely excited to develop a GRS–IBS project that would turn the technology from innovative to conventional construction,” says Javier Arroyo-Rosario, PRHTA’s deputy executive director for infrastructure. “We were pioneers in the early nineties, implementing ABC techniques when the Baldorioty De Castro Avenue bridges were constructed in 72 hours [total] on one of the busiest roads in Puerto Rico. At that time PRHTA was extremely successful, but the technology was not implemented again.” PRHTA is making sure this time will be different.
According to FHWA’s Geosynthetic Reinforced Soil Integrated Bridge System Synthesis Report (FHWA-HRT-11-027), GRS–IBS is easy to design and cost-effective to construct. The system can be built in variable weather conditions with readily available labor, materials, and equipment and can easily be modified in the field. Also, this method is ideal for single-span structures. PRHTA chose to implement the technology for all these reasons.
PRHTA identified adjacent twin bridges that presented an excellent opportunity to implement the GRS–IBS technology. Design of the project began in 2011, which started PRHTA on a quest to incorporate the innovation into its standard construction practice.
How It Started
The original bridges 1121 and 1122 were twin slab structures constructed in 1970 on PR–2, a road in the Puerto Rican component of the National Highway System. PR–2 facilitates the movement of people and goods between the municipalities of Mayagüez and Ponce, the biggest economic drivers of the western and southern regions, respectively.
Both superstructures were showing signs of deterioration, which prompted the need for replacement. Inspectors recorded the condition rating of the slab and beams on bridge 1122 as “poor” because of cracking. In fact, prior to the replacement project, PRHTA temporarily supported the bridge by installing a steel frame at one of the piers. For bridge 1121, the condition rating was listed as “fair” for the slab and beams.
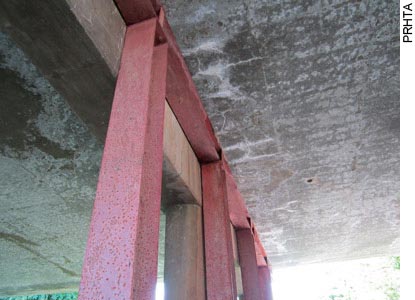
Each of the existing bridges had a three-span configuration, each with a total length of nearly 80 feet (24 meters). But, PRHTA’s engineers determined each structure could be replaced with a single span bridge of approximately 36 feet (11 meters). The single span of each new bridge would continue to serve as a travel way for cattle passing underneath, accomplished by building the new GRS abutments to the edge of the existing piers.
The location and traffic conditions made the bridges ideal candidates for implementing the GRS–IBS technology. Why? The conditions offered four major advantages. First, the short single-span replacements were ideal for Puerto Rico to try GRS–IBS for the first time. (Now that the project has proven successful, PRHTA is ready to implement the technology in a broader manner and in bridges of greater spans.) Second, PRHTA could use void slabs (prestressed elements with longitudinal circular voids) directly on top of the GRS–IBS, eliminating the need to cast a concrete leveling pad. Third, no water runs under the bridges, which meant that no special considerations had to be made to address scour. PRHTA officials decided that for the agency’s first project, the absence of water would make the process easier and eliminate the possibility of complications due to flooding, which is typical in Puerto Rico throughout the year. (In subsequent projects, such as bridge 1828 on PR–140 in Barceloneta, PRHTA is using the technology to replace structures over water.) Lastly, the high average daily traffic volume on the bridges required the use of an accelerated bridge construction technique such as GRS–IBS.
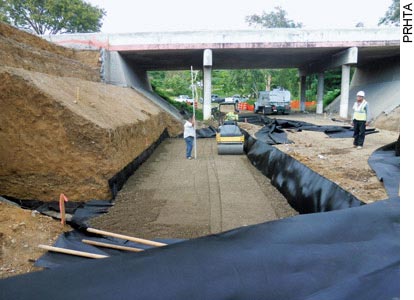
To obtain funding for the project, PRHTA submitted a request for consideration under FHWA’s Innovative Bridge Research and Deployment Program, which awarded the agency a $370,000 grant.
“The use of GRS–IBS as promoted through the Federal Highway Administration’s EDC initiative has seen tremendous growth over the past 3 years — from a few projects in 2 States to more than 150 projects in more than 35 States around the country,” says Daniel Alzamora, senior geotechnical engineer at the FHWA Resource Center in Colorado. “Although the majority of the projects continue to be on the local road system, there are a few bridges on interstates that are utilizing this technology to reduce project delivery time and cost. The replacement of bridges 1121 and 1122 on Puerto Rico’s PR–2 is one of those projects.”
Although the twin bridges would become the third GRS–IBS project constructed on the National Highway System, bridges 1121 and 1122 carry higher average daily traffic (ADT) counts than the previous projects — more than 40,000 including 11 percent truck traffic.
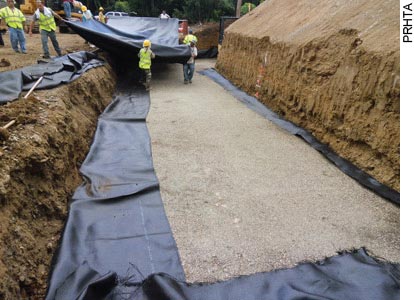
Preliminary Design
As part of the implementation of GRS–IBS technology, PRHTA coordinated a workshop with FHWA to introduce the technology to the Puerto Rican staff, consultants, contractors, and suppliers. The workshop took place at PRHTA’s main offices in San Juan from February 7–9, 2011, and included a site visit to bridges 1121 and 1122. FHWA’s Alzamora led the workshop, along with Jennifer Nicks, a research geotechnical engineer, and Michael Adams, a research civil engineer, both based at FHWA’s Turner-Fairbank Highway Research Center. The workshop focused on the design and constructability aspects of the technology.
By the end of the workshop, participants had learned about the major components of GRS–IBS and the benefits and limitations of using the technology in highway applications. Participants also covered suitable site conditions and rules for proper construction, as well as the steps required to design a GRS–IBS structure.
During the workshop, the instructors provided a Microsoft® Excel® spreadsheet for the preliminary design of the GRS–IBS structure, based on FHWA’s Geosynthetic Reinforced Soil Integrated Bridge System Interim Implementation Guide (FHWA-HRT-11-026). PRHTA used this spreadsheet as the basis for design of its GRS–IBS structures. While the limits and restrictions in the spreadsheet provide for construction of durable and secure systems, PRHTA tailored some aspects of the spreadsheet to meet its own needs. Some of the modifications are as follows.
User interface. PRHTA added a graphic interface to help users understand the input and output data. The inclusion of graphs and diagrams helps users visualize each change made during the design process, which often requires verification and comparison of multiple configurations of the GRS–IBS components.
Seismic criteria. In Puerto Rico the seismic horizontal peak ground acceleration varies approximately between 0.28g and 0.34g (where “g” is the gravity standard acceleration). Because the spreadsheet considered only static loading, PRHTA modified it to include AASHTO seismic criteria.
Global stability criteria. When a wall structure and adjacent soil mass move, it is called a global stability failure. Global stability includes rotational or compound failures, which differ from a sliding or overturning failure. PRHTA added the stability criteria as a method of preliminary assessment of the external stability of the GRS before entering the model into a slopes stability analysis program. Inclusion of this analysis optimizes the design process, avoiding the need to perform several iterations and geometric changes due to the global stability criteria. Engineers developed such preliminary analysis using the Bishop’s Modified Method of analysis. Finally, the design was validated through the use of proprietary software that performs global stability analysis.
Special Design Provisions
PRHTA developed Special Provision 983 – Geosynthetic Reinforced Soil Integrated Bridge System in coordination with the FHWA Resource Center. The provision allows for the use of both well-graded and open-graded backfill. Open-graded backfill is used when engineers expect that the GRS–IBS structure might be submerged during flood events. The provision also allows for the use of solid and hollow blocks. The bridge owner determines which type of block to use.
PRHTA also developed another document, Special Provision Specification 975 – Bridge Monitoring and Instrumentation System, for the instrumentation and monitoring of the bridges using fiber-optic sensor technology. The monitoring system is of particular importance to PRHTA because it enables comparison between design assumptions and theory, and the actual behavior of the GRS–IBS structure.
Some of the most important aspects of Special Provision Specification 975 include the following.
Redundancy. Fiber-optic sensors are a high-accuracy technology and provide redundancy because the sensors have two fiber-optic cables. One is connected to the data acquisition equipment, and the other is a spare cable in case the first one fails. This technology allows for long distances from the point at which the sensors are installed to the location of the equipment for collecting the data.
Extended life. The fiber-optic monitoring system has a long lifespan because it does not suffer deterioration due to moisture or temperature changes, and it is immune to electrical discharges.
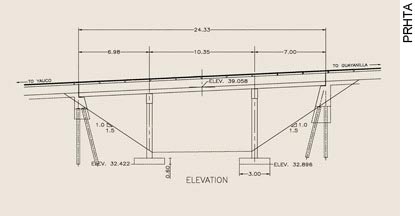
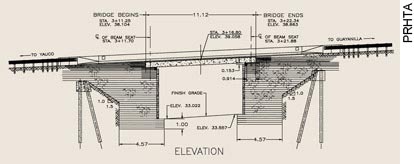
High precision. The data acquisition equipment and sensors do not require calibration because they do not have analog components. In other words, all the electronics are digital and more accurate because they are not affected by electrical noise.
Requirement of previous experience. To ensure the accuracy of the data, the technical staff performing the installation of the fiber-optic system had to have at least 5 years of experience in the instrumentation of bridges with this technology and have obtained the relevant certifications.
Bridge 1121, the second bridge PRHTA replaced, was instrumented with 20 pressure cells (10 on each abutment in the GRS mass) to measure the superstructure’s vertical pressure distribution, and 16 geosynthetic fiber-optic sensors (8 on each abutment) in the integration zone and GRS mass) to measure stresses and strains. A system of solar panels supplies power for the monitoring system, and data transmits in real time from the bridge to a central server located at the PRHTA main building. From there, PRHTA and partners can access the data for analysis of bridge behavior. Data collection on the completed bridge began in summer 2014.
Determining a Backfill
Since the bridges do not cross water, PRHTA officials decided in the design stage to use a well-graded, rather than an open-graded, backfill for the GRS–IBS structure. The designers used as guidance a gradation similar to the one presented in section 3.3.1.1 Well-Graded Backfill in the Interim Implementation Guide.
The contractor was required to provide a backfill material to meet the following specifications:
- Maximum aggregate size limited to 2 inches (5 centimeters)
- Twelve-percent maximum fines passing number 200 sieve
- Friction angle of at least 38 degrees
- Plasticity index equal to or less than 6
- A magnesium sulfate loss of less than 30 percent after four cycles (or a sodium value less than 15 percent after five cycles) per AASHTO T-104
Adding a Reinforced Soil Foundation
The original set of plans did not include construction of a reinforced soil foundation because the plans called for the GRS to be constructed directly on top of the existing piers after the columns were cut off at the top of the footings. The plans did specify, however, increasing the size of the existing separate footings to convert them to a single continuous foundation.
According to the original plans, the tops of the footings of the existing bridge were just below grade. But when the contractor began excavating and cut the columns on bridge 1122, the workers found that the actual depth of the top of the footing was 5 feet (1.5 meters) below ground level. Because of this change in elevation and to avoid having a GRS with a height considerably different from the initial design, the contractor constructed a 5-foot (1.5-meter) reinforced soil foundation on top of the existing foundation.
Moving Blocks: Are They Alive?
From the start of the construction process, the contractor and PRHTA inspection personnel noticed that the concrete blocks for the abutments tended to move forward when the compaction roller was in use. Even by limiting compaction to 3.3 feet (1 meter) behind the blocks, the displacement was noticeable at more than 1 inch (2.5 centimeters).
This displacement slowed down the construction process because the crew had to use rubber hammers to move the blocks back after each compaction layer. As a result, PRHTA officials requested FHWA’s assistance in resolving the issue of block movement. It was determined that the primary issue was the excessive compaction of the well-graded fill material, which was drier than the optimum moisture content. The fact that the hollow-core facing blocks were lighter than normal might have been a contributing factor as well.
For the second bridge (bridge 1121), PRHTA and the contractor agreed to replace the hollow-core blocks with heavier 66-pound (30-kilogram) solid blocks and to adjust the number of passes to achieve the specified compaction. These measures proved effective, and bridge 1121 showed no displacement in the blocks during the compaction process.
Timeline of the Bridge 1122 Replacement
The total construction time for the replacement of bridge 1122, after demolition of the existing structure, was 45 days. If the project had called for conventional concrete cast-in-place abutments, it would have taken an estimated 120 to 150 days for the construction of the abutments. The timeline for the various stages of work on bridge 1122 was as follows.
- Excavation and construction of reinforced soil foundation for both abutments: 10 days
- GRS construction for both abutments: 18 days
- Installation of void slabs, transverse post-tensioning, and longitudinal joint grouting: 2 days
- Approach embankments and IBS for both abutments: 5 days
- Parapets (protective walls of earth): 8 days
- Asphalt installation (including water membrane on top of void slabs): 2 days
Bridge 1121 was constructed faster than bridge 1122 because the contractor was more familiar with the technology. However, because of the installation of the monitoring system at both abutments and a technology showcase held at the project site, it took the same number of days (45 days) to complete the second bridge. PRHTA officials estimated that construction of the second bridge without the monitoring system would have taken between 35 and 40 days. Both bridges are now open to traffic.
Lesson Learned
PRHTA and the construction team learned a significant lesson during construction of the GRS–IBS for bridge 1122. PRHTA officials realized that for well-graded materials that are drier than the optimum moisture content, the compaction process can affect the alignment of the hollow blocks because a 95-percent compaction is required. Therefore, the loose materials caused increased forces on the blocks, which made them shift laterally. In the case of open-graded material, the compaction requirement is to compact to nonmovement or no appreciable displacement, and to assess with visual inspection. Open-graded material is also easier to source in Puerto Rico and faster to place and compact.
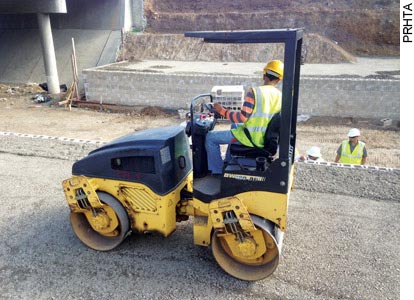
As a result, PRHTA made two changes in Special Provision 983 – Geosynthetic Reinforced Soil Integrated Bridge System. First, although the use of well-graded backfill could be acceptable in applications where the GRS will not be submerged, only open-graded material is permittted. This determination is based on the particular characteristics and availability of the backfill materials in Puerto Rico. Second, the provision now specifies the use of solid blocks with a minimum weight of 66 pounds (30 kilograms) for the facing of the abutments.
As noted earlier, PRHTA held a showcase in April 2014 during the construction of bridge 1121 with an attendance of more than 80 representatives from PRHTA, local designers, contractors, material suppliers, and transportation personnel from the U.S. Virgin Islands. The showcase had a morning session during which presenters discussed the technology and introduced participants to its benefits. In the afternoon session, participants visited the project site and were able to see the backfill compaction and instrumentation process.
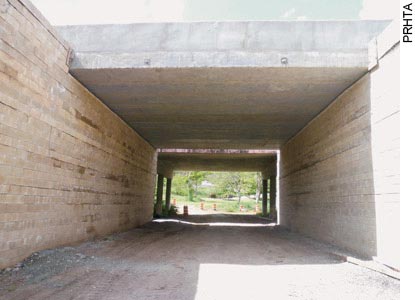
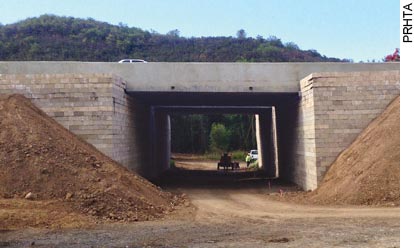
“This project has been very successful thanks to the commitment of everyone, from the contractor to FHWA and PRHTA staff, including the designer and the project inspector,” says Yaritza Cordero, PRHTA construction area project administrator. “We all have developed a great partnership that has helped to overcome the few unforeseen situations [we] encountered during construction. We are confident that this technology has arrived to stay, and that it will signify a great benefit for the public by reducing the construction time for many future projects.”
Stay tuned, as a future issue of Public Roads will discuss the instrumentation process during construction of bridge 1121.
Héctor Laureano-Pagán, P.E., was the bridge program manager with PRHTA, where he worked from 1997 to May 2014 in areas related to bridge design, construction, preservation, and management. Laureano-Pagán is now the bridge engineer in the FHWA Mississippi Division Office responsible for the oversight of State compliance with Federal regulations. He has a bachelor’s degree in civil engineering from the University of Puerto Rico.
José R. Rodríguez-Pacheco is the structural engineer and landslide correction specialist with PRHTA since 2000. He has more than 13 years of experience in planning, design, inspection, and construction of structures for public transportation. Rodríguez-Pacheco has a bachelor’s and a master’s degree in civil engineering from the Polytechnic University of Puerto Rico.
The authors would like to thank PRHTA staff members Francisco Padua, Yaritza Cordero, and Ivelisse Pérez, as well as University of Puerto Rico at Mayagüez professor and director of the Local Technical Assistance Program, Benjamin Colucci, and his staff who worked to make the project a success. The authors also want to recognize the valuable contributions of FHWA Puerto Rico Division Office staff members Maribel Pérez, Evelyn Colón, Alvin Gutierrez, Eddie Rivera, Carlos Machado, and Martin Knopp, as well as Jeffrey Ger and Derek Soden from the FHWA Florida Division Office.
For more information, contact Héctor R. Laureano-Pagán at 601–965–7330 or hector.laureano@dot.gov