A Better Design for Box Culverts?
FHWA and the South Dakota Department of Transportation recently partnered to study the effects of inlet geometry on water flow in cast-in-place and precast structures.
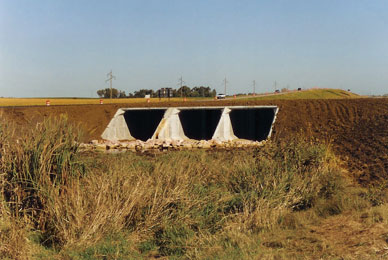
(Above) The South Dakota Department of Transportation (SDDOT) installed this triple-barrel box culvert, made from precast concrete, under a median crossover on Interstate 29 in Minnehaha County, just north of Sioux Falls, SD. FHWA and SDDOT recently conducted a study of various inlet section configurations for box culverts with the goal of updating industry design software and coefficients. Photo: Corey Haeder, Cretex Concrete Products West, Inc. |
Understanding hydraulics and hydrology is a necessity for designing drainage structures such as culverts that control the flow of water near highway infrastructure. The size and shape of a culvert not only determine the structure's effectiveness, especially during extreme weather events such as major floods and washouts, but they also significantly affect the overall construction costs of a project.
"Culverts that are designed using older, more conservative inlet coefficients tend to be oversized," says Mark Clausen, division bridge engineer with the Federal Highway Administration's (FHWA) South Dakota Division. Oversized culverts equate to higher costs than are necessary to do the job effectively, especially for construction materials such as concrete.
"This extra expense means that there will be fewer construction projects for State DOTs [departments of transportation] and county highway agencies," Clausen says. "In these times of budget constraints, getting more efficient designs and making the tax dollar go as far as possible is the goal for States and counties."
State DOTs are interested in culvert hydraulics because they install a large number of culverts every year. Based on 2001 Form FHWA-47 data (which catalogs materials and labor used by contractors on highway construction projects involving Federal funds), more than 769,443 meters (2.5 million linear feet) of pipe materials between 45 centimeters (18 inches) and 560 centimeters (220 inches) in diameter were installed per year on Federal-aid projects that cost more than $1 million each. That equates to roughly 7,700 kilometers (4,780 miles) over a 10-year period. Although this statistic includes all pipes, culverts represent a high percentage of the total in this size range. Further, this statistic does not include field-cast concrete culverts, but it does reflect the order of magnitude of culvert installations in the United States.
To design and analyze culverts, most State DOTs rely on FHWA's Hydraulic Design Series (HDS-5), Hydraulic Design of Highway Culverts (FHWA-IP-85-15), and the companion computer program, HY8 Culvert Analysis (Version 6.2). First published in 1985, HDS-5 is based primarily on research that was sponsored by the Bureau of Public Roads (the predecessor to FHWA) in the late 1950s and early 1960s and industry-supported research conducted during the 1970s.
Since the initial publication of HDS-5, researchers, especially in the precast industry, have developed a number of alternative configurations for box culverts, which are usually square or rectangular reinforced concrete structures with single or multiple openings. New culvert configurations and designs raise questions about the effectiveness of subtle differences or changes in the geometry or configuration of the inlet (edges of the culvert). To answer some of these questions, FHWA and the South Dakota Department of Transportation (SDDOT) partnered to conduct a study in the FHWA Hydraulics Laboratory located at the Turner-Fairbank Highway Research Center (TFHRC) in McLean, VA.
In South Dakota, as in other States, engineers have the option of using precast or cast-in-place concrete for box culverts. Precast inlets generally have straight (zero-degree) wingwalls, while field-cast inlets may feature straight or flared wingwalls. (In a straight inlet, the wingwalls extend straight out from the end of the barrel section. With a flared inlet, the wingwalls flare outward, creating a sort of funnel shape before the barrel section.) According to Clausen, the current inlet coefficients used in computer models frequently specify larger openings for the precast options than needed, leading to oversized and conservative designs for straight inlets.
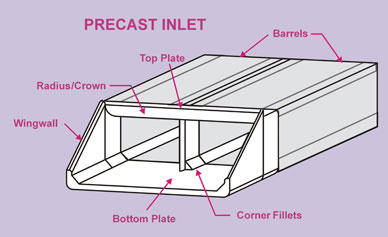
These box culvert diagrams show the key components of double-barreled precast (left) and field-cast (right) inlets, including the top and bottom plates, crowns, wingwalls, and corner fillets. A primary difference between the two inlets is that the precast type features a straight wingwall, whereas the field-cast version has a flared wingwall, which helps the culvert act more like a funnel. Source: FHWA. |
The FHWA-SDDOT research project, Effects of Inlet Geometry on Flow Capacity of Single and Multiple Barrel Box Culverts, therefore examined the coefficients for straight and flared inlets. "The gap between the coefficients for the two inlet types is not as large as once thought," Clausen says. "The data from this study soon will be incorporated into current hydraulic design software, and box culvert designs should become more cost effective and efficient in the future."
Research Considerations
The precast industry can produce a variety of edge treatments for culverts once the forms are fabricated. Therefore, the first factor that the research team considered was the effect of streamlining the bevels on the top plate and wingwalls at the culvert entrance. The researchers expected to find that by streamlining the edges of straight wingwalls they could yield enough hydraulic gain to compare favorably with the hydraulic performance of flared wingwalls, which are common among cast-in-place installations in the field.
Other factors investigated in the study include corner fillets (fabricated corners filled slightly to avoid concentrating stress in sharp turns), multiple barrels (a culvert unit composed of two or more barrels adjacent to each other), extended inner walls, span-to-rise ratios, skewed crossings, submerged and unsubmerged inlets, and two different barrel slopes. The factors were tested under a wide range of flow conditions to determine how they affect the design coefficients used to evaluate culvert performance for both outlet control and inlet control situations. The design coefficients derived in this study were the entrance loss coefficients, which are the numbers multiplied by the velocity head to estimate the entrance energy loss for a culvert operating in outlet control where the headwater depth is influenced by tail water and friction in the culvert as well as the entrance loss. A lower entrance loss corresponds with a lower headwater at the upstream side of the highway embankment. The design coefficients are a set of regression coefficients relating headwater depth to discharge intensity for each inlet configuration for culverts operating in inlet control, where the headwater depth is influenced by the inlet geometry but not by friction in the culvert or the tail water.
Although engineers traditionally treated culverts with multiple barrels as a combination of single-barrel units, the research team sought to challenge the adequacy of that assumption. Intuitively, the researchers expected that a multiple-barrel installation might perform slightly better than a number of adjacent single-barrel culverts because the inner barrels do not have much flow contraction.
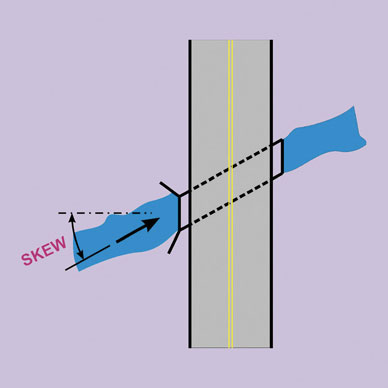
The researchers tested skewed headwalls for their effectiveness in controlling flow through skewed highway alignments such as the one shown in this illustration. The study did not, however, test culvert alignments skewed to stream alignments. Source: FHWA. |
The inner walls of a multiple-barrel installation are usually flush with the headwall, but the researchers questioned whether there might be an advantage to extending the inner walls onto the approach apron, just as the wingwalls are extended. "The precast industry tends to use combinations of single-barrel and double-barrel components to make triple- and quad-barrel installations," says Corey Haeder, chief engineer with Cretex Concrete Products West, Inc. By placing the two culverts side by side, the inner wall becomes thicker. He adds, "That practice leaves one double-thick inner wall, which is a detriment, but to what degree?"
Further, because highway alignments are not always perpendicular to streams, culverts should be skewed to the highway embankment, as opposed to attempting to change the direction of the stream. Although the authors have observed a number of installations where the culvert did change the flow direction, the tests conducted in this study were limited to cases where the highway alignment and culvert wingwalls, but not the culvert barrel, were skewed to the flow direction.
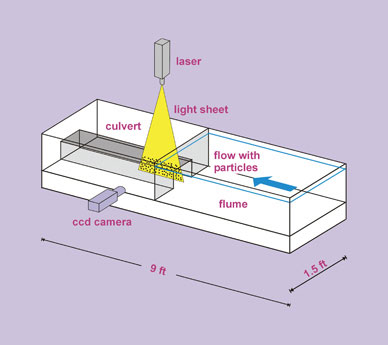
This diagram shows components of the miniflume and setup for particle image velocimetry. From the right-hand side, the particle-laden water flows from the flume and enters the culvert. A laser mounted above the flume directs a sheet of light down through the culvert, where a chargecoupled device (CCD) camera collects information about the velocity of the entrained particles passing through the culvert. Source: FHWA. |
Setting Up the Experiment
Researchers conducted approximately 700 tests at the TFHRC Hydraulics Laboratory, with the physical modeling for the culvert study occurring in two phases. The first set of experiments optimized the bevel edge of the wingwalls and top edge using two-dimensional particle image velocimetry (PIV) in a 2.75-meter (9-foot)-long and 0.46-meter (1.5-foot)-wide flume, an artificial channel for measuring water flow. This technology uses a laser and particles of silver-coated and hollow glass spheres to make the flow visible. Cameras are used to measure instantaneous velocity vectors in a flow field.
The researchers tested several bevel edge conditions, including straight top bevels (square edge), beveled edge (45 degree bevel), and radius top bevels. The criterion they used to determine the best bevel performance was the contracted distance outside the viscous boundary layer, or the effective flow depth at the vena contracta (where the flow depth is lowest inside the barrel section). The researchers used integrated streamlines, which indicate the speed and direction of flow, to visualize the contracted area (the area where water flow narrows from a wider flow) inside the culvert.
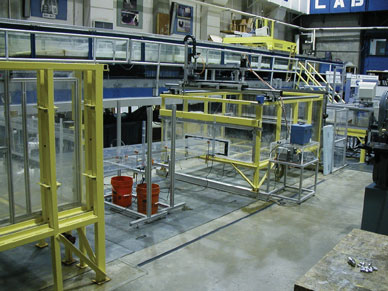
This photo shows the culvert setup in the FHWA Hydraulics Laboratory, with the model barrel between the head and tail boxes. |
A second phase of experiments consisted of a culvert setup with a head box measuring 2.4 meters (8 feet) long by 2.4 meters (8 feet) wide by 1.2 meters (4 feet) high, and a tail box measuring 2.4 meters (8 feet) long by 1.8 meters (6 feet) wide by 0.9 meter (3 feet) high. The boxes were connected by Plexiglas® model culvert barrels with various inlet configurations. Plexiglas was used because it has similar friction to that of concrete in the model scale, yet unlike concrete would enable researchers to view the behavior of the water flow. The inlet models were designed as modular clip-in parts (wingwalls, center walls, and top plates) that could be changed easily to test various configurations. Two sets of model fillets-12.7 millimeters (0.5 inch) and 25.4 millimeters (1 inch)-were fabricated using polyvinyl chloride (PVC) material.
Electronic pressure sensors installed in the floors of the head and tail boxes measured the headwater and tail water depths. The researchers installed as many as 40 pressure sensors in the culvert barrels to measure the hydraulic and energy grade lines that were necessary to compute loss coefficients. An automated tailgate (a gate that regulates water flow depth in the flume) at the downstream end of the tail box helped maintain control of the tail water. The barrels were tested for two different slope settings. The steep slope (3 percent) accounted for inlet control conditions. The flat slope (0.7 percent) correlated with outlet control conditions and low tail water depths.
a)
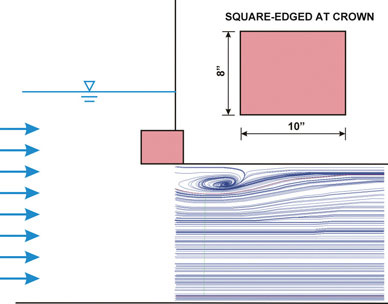
b)
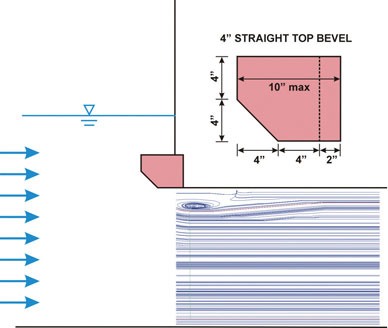
c)
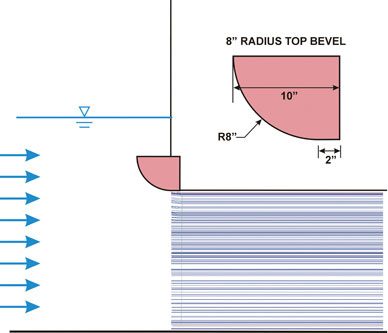
This series of figures illustrates the effective flow depth at vena contracta for three bevel patterns: (a) squareedged bevel at crown, (b) 102-millimeter (4-inch) straight top bevel, and (c) 203-millimeter (8-inch) radius top bevel. The first two patterns tended to generate eddies at the top of the flow column, indicating disrupted flow. The 8-inch radius top bevel, however, offered the optimal geometric orientation, as illustrated by the undisturbed flow pattern. Source: FHWA. |
Test Results
Postprocessing of PIV results provided streamlines that the researchers could interpret visually to show the shape that produces the maximum effective flow depth at the vena contracta. This shape is likely to have the least headloss (loss of energy in a hydraulic system) when incorporated into the inlet geometry, meaning water will flow through faster and more effectively because of the shape of the bevel. The results show that the 203-millimeter (8-inch) rounded bevel edge produces the optimal geometric configuration.
The PIV miniflume tests helped identify the best shape, but the researchers also wanted to know how much gain the shape produced in the hydraulic performance. To answer this question, they tested two types of models: precast and cast-in-place. The precast models were fabricated with the optimum radius on the top plate and rounded bevels with a 102-millimeter (4-inch) radius on the wingwall edges. The field-cast models featured SDDOT's standard straight bevel (45 degrees) on the top plate and no bevels on the wingwall edges. The researchers conducted additional tests using a model of the closest HDS-5 inlet, which has wingwalls with a zero-flare angle and no bevels on either the top plate or the wingwalls.
a)
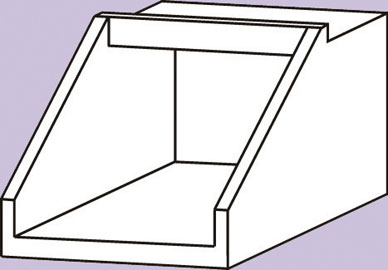
HD-5 Inlet
0° flared wingwalls
Square-edged at crown
No wingwall bevel
No corner fillets
b)
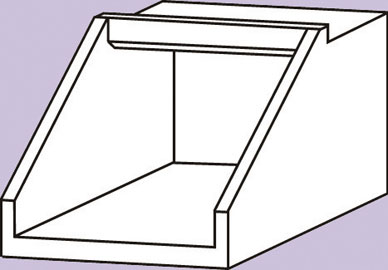
0° flared wingwalls
4-inch straight top bevel
No wingwall bevel
No corner fillets
c)
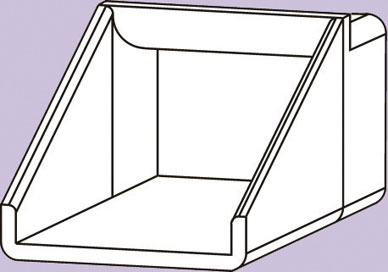
0° flared wingwalls
8-inch radius top bevel
4-inch radius wingwall bevels
No corner fillets
The researchers studied the effects of bevels on three types of models: (a) an HDS-5 inlet, (b) a field-cast model, and (c) a precast model. Source: FHWA. |
Based on their findings, the researchers concluded that there is almost no gain due to edge bevel shapes for unsubmerged inlet control flow, which is understandable because the top edge with the primary bevel is not exposed to the flow. The gain shows up in the submerged section and increases to approximately 10 percent less headwater with the 203-millimeter (8-inch) radius top plate at the highest discharge intensity tested. The precast inlet with the optimum top plate bevel proved to be the best performer when the inlet was submerged, because the streamlined curve of the bevel provides a better path for the water flow. Although the researchers did not attempt to verify their results using actual field data during this study, they contend that the results correlate well with previous studies and coefficients currently in HDS-5.
To understand the effects of using multiple barrels, the researchers sought to answer two questions. First, is it reasonable to assume that multiple barrels control flow in the same way as multiples of a single-barrel culvert with the same type of inlet? Second, is there a hydraulic advantage to extending the center walls onto the apron?
After testing a number of field-cast and precast models, the researchers found almost no difference in the performance of multiple barrels and single-barrel culverts for unsubmerged flow during inlet control conditions. They noted, however, that a slight advantage exists for multiple barrels compared to single barrels for submerged flow for the field-cast models and a significant hydraulic advantage for the precast models with the optimum bevel on the top plate, especially for headwater depths greater than 1.5 times the culvert height. All of the inlet control results indicate that the common practice of using single-barrel coefficients for multiple-barrel culverts is conservative for inlet control conditions. In other words, the same coefficient can be used for both single barrels and multiple barrels for unsubmerged inlet control.
The researchers found that the outlet control results were somewhat inconsistent. The values for the entrance loss coefficient for the field-cast models with straight wingwalls were almost identical for the single-, double-, triple-, and quad-barrel models and averaged 0.52. Similarly, the outlet control coefficient values for the field-cast models with 30-degree flared wingwalls were close to an average value of 0.31 for the single-, double-, triple-, and quad-barrel models. These results support the practice of using the single-barrel coefficients for multiple-barrel analyses for outlet control, as well as inlet control, conditions for field-cast box culverts.
For the precast models, however, this is not the case. The average entrance loss coefficient increased from 0.33 for the single barrel to 0.49 for the double barrel to 0.54 for the triple barrel to 0.59 for the four-barrel model, suggesting that the efficiency decreases with the number of barrels for precast box culverts operating in outlet control conditions. The researchers explain the decrease in part by the double-wall thickness that results from setting two sections side by side to yield the triple- and four-barrel configurations, but they could not explain why the double barrel showed a higher loss coefficient than the single barrel.
The results for inlet and outlet control showed no hydraulic advantage or disadvantage to extending the inner walls of multiple-barrel culverts onto the apron. Further, the researchers noted a significant increase in headwater for a given discharge intensity for skewed headwalls, which are sometimes used to accommodate highway alignments that are not perpendicular to the stream flow.
Significant Findings
All of the inlets tested in the study were variations of inlets covered in HDS-5, with different combinations of entrance improvements. The following are among the significant findings:
- Based on the PIV flow visualization technique developed in the TFHRC laboratory, the researchers determined that the optimum edge treatment for the top plate, or crown, of a culvert is a rounded radius of 203 millimeters (8 inches), which happens to be the full thickness of the top plate for the models tested.
- A distinct difference in performance exists between square-edged crowns, beveled crowns, and rounded crown plates for box culvert models with straight wingwalls under submerged conditions. The rounded crown plate performed the best under submerged inlet conditions; the square edge performed the worst.
- Multiple barrels had a slight, but negligible, hydraulic advantage over single-opening culverts for the inlet control tests, except for the precast models with submerged inlets and headwater depths greater than 1.5 times the culvert height, where there was a significant advantage over the multiple barrels. However, the researchers noted that highway agencies seldom design for headwater depths greater than 1.5 times the culvert height. Multiple-barrel models with flared wingwalls, on the other hand, were slightly less hydraulically efficient for the outlet control tests where the entrance loss coefficient varied from 0.26 for the single-barrel models to 0.34 for the worst multiple-barrel model. The researchers noted, however, that the entrance loss is a relatively small part of the total losses controlling the headwater for culverts operating under outlet control. Further, they concluded that it is reasonable to use the single-barrel coefficients for multiple-barrel culverts.
- Wide span-to-rise models acted similarly to multiple barrels, except there was a slight hydraulic disadvantage (rather than advantage) in the coefficients for the wide-span models compared to the 1:1 span-to-rise models for both the inlet control tests and the outlet control tests.
- There was no hydraulic advantage or disadvantage to extending the inner walls of multiple-barrel culverts onto the apron.
- Skewed headwalls have a detrimental effect on culvert hydraulics.
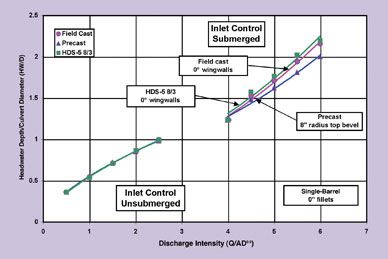
This graph shows inlet control performance curves for HDS-5, field-cast, and precast inlets. The y-axis plots headwater depth divided by the culvert diameter, and the x-axis shows the discharge intensity for the three models-one with the optimum top plate, one with the straight cut bevel, and one with the square edges. The lower curves in the chart indicate better performance, meaning that the inlet operates with less headwater for a given discharge intensity. The inlet with the optimum top plate bevel was clearly the best performer when the inlet was submerged. When the inlet was unsubmerged, all three curves were on top of one another, indicating that the bevels made almost no difference. Source: FHWA. |
A big concern for SDDOT was not only achieving the research results but also incorporating the results into culvert design software. To facilitate transferring the data to the software, the researchers presented inlet and outlet control loss coefficients in a format consistent with HDS-5 and developed fifth-order polynomials (regression curves to fit the experimental data) to be coded into the design software. The authors understand that many users would prefer to use the design software on a Windows® operating system and have the architecture modified to facilitate incorporating new test results when they become available from reliable research laboratories.
The timing of revisions to HDS-5 and the design software will be driven by selected contributions from this study as well as a major parallel study sponsored by the National Cooperative Highway Research Program (NCHRP) at Utah State University, where researchers are investigating a number of environmental considerations that engineers frequently encounter in culvert design.
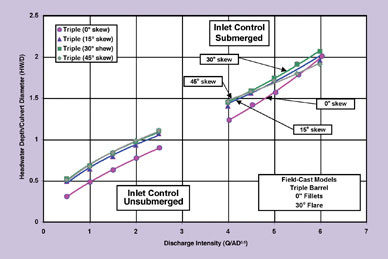
Inlet control comparison for multiple-barrel, field-cast skew tests with 30-degree flared wingwalls. The graph is a plot of headwater depth divided by culvert diameter on the y-axis versus discharge intensity on the x-axis for the four models with various headwall alignments skewed to the stream flow direction. The lower curves in the chart indicate better performance, meaning that the inlet operated with less headwater for a given discharge intensity. The zero skew angle clearly was the best performer for both submerged and unsubmerged flow. The curves for the other skew angles tested-15, 30, and 45 degrees- could be merged into a single curve without much loss in accuracy. Source: FHWA. |
Kornel Kerenyi is a hydraulic research engineer in the FHWA Office of Infrastructure Research & Development (R&D). He coordinates FHWA hydraulic and hydrology research activities with State and local agencies, academia, and other partners and customers. He also comanages the FHWA Hydraulics Laboratory. Previously he was a research engineer for GKY and Associates, Inc. and supervised the support staff in collecting and analyzing the data for this study. Kerenyi holds a Ph.D. in fluid mechanics and hydraulic steel structures from the Vienna University of Technology in Austria.
J. Sterling Jones is a hydraulic research engineer in the FHWA Office of Infrastructure R&D. He manages the FHWA research program in hydrology and hydraulics and comanages the Hydraulics Laboratory. He oversaw data collection and analysis and the reporting of results for this study. He is a registered professional engineer in Virginia.
Kevin Goeden is the bridge design engineer for the SDDOT Office of Bridge Design. He served as a technical panel member for the research. Goeden has been involved in highway structure design activities in the Office of Bridge Design for more than 23 years and is the engineering supervisor in charge of the bridge design and hydraulics sections. He holds a B.S. in civil engineering from South Dakota State University and is a registered professional engineer in South Dakota.
Richard Phillips is the bridge hydraulics engineer for SDDOT. He manages hydrologic and hydraulic design of large culverts and bridges for State route projects and reviews hydraulic design for federally funded local government projects. He provided input into developing the statement of work for this study, participated in project meetings, and reviewed reports. He collaborated with Sterling Jones in a joint presentation on preliminary results from the study at the 2004 FHWA National Hydraulic Engineering Conference in Asheville, NC. He is a registered professional engineer in South Dakota.
Paul Oien is a project engineer with the SDDOT Office of Research. He served as the project monitor for this study. He coordinates SDDOT research activities with other State agencies, construction industry representatives, manufacturing representatives, promotion boards, and other stakeholders to ensure that projects are completed as stated in research proposals. He holds a B.S. in civil engineering from the South Dakota School of Mines and Technology.
To access the HDS-5 report, please visit https://www.fhwa.dot.gov/engineering/hydraulics/pubs/hds4intr.pdf. For more information on this study, see the laboratory report, Effects of Inlet Geometry on Hydraulic Performance of Box Culverts, which is scheduled to be published in 2006.