Ultrasonic Inspection of Bridge Hanger Pins
Adapted from TRB Paper No. 00-0918 with the same title by Graybeal, Walther, and Washer. The original paper was prepared for the 79th Annual Meeting of the Transportation Research Board on Jan. 9-13, 2000, and was published in Transportation Research Record (TRR) No. 169,, Maintenance and Management of Bridges and Pavements, July 31, 2000, pages 19-23.
In June 1983, a failed hanger pin initiated the tragic collapse of one span of the Mianus River Bridge on the Connecticut Turnpike near Greenwich, Conn. This incident resulted in the deaths of three motorists. Following the collapse, there was an immediate increase in interest in the inspection and condition evaluation of bridge hanger pins.
Ultrasonic inspection is one of the most reliable methods used to inspect hanger pins, and it has become the primary method of performing a detailed inspection of an in-service hanger pin.
This article describes a study conducted by the staff of the Federal Highway Administration's (FHWA) Nondestructive Evaluation Validation Center (NDEVC) to determine the reliability of contact ultrasonic techniques in the field to accurately locate defects in hanger pins. The study examined and compared two ultrasonic techniques. The first technique involves testing in-service pins using a contact ultrasonic method. This type of inspection would generally be used during an in-service inspection.1 The second technique involves testing decommissioned pins through a noncontact ultrasonic method using an immersion tank. While this type of inspection is not practical for field use, it does provide highly repeatable and reliable results that are intended to help verify the contact ultrasonic inspection methods.
This article provides some background information regarding hanger pins in general, as well as the hanger pins studied in this paper, and it discusses the field ultrasonic technique, including methods, results, and limitations; the immersion tank ultrasonic testing, also including methods, results, and limitations; comparisons between the two testing methods; and conclusions.
Background
Hanger pins are the structural elements connecting the suspended span of the bridge to the fixed cantilever arm of that same bridge. A diagram of a pin-and-hanger connection is shown in figure 1. Photographs of one of the hanger pins examined for this study are shown in figures 2 and 3. Note that one of the exterior faces of the pin can be seen in figure 2 and that the exterior face and the shear planes are identified in figure 3. The exterior face is defined as either of the ends of the pin that are visible while the pin is still in the pin-and-hanger connection. The exterior faces are the only surfaces through which ultrasonic pulses can be transmitted while the pin is in situ.
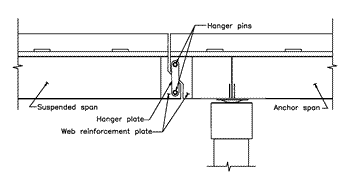
The primary function of a pin-and-hanger connection is to allow for longitudinal thermal expansion and contraction in the bridge superstructure. These connections are designed to support the transfer of shear forces from the suspended span into the anchor span. As long as the connection is operating properly, neither shear forces from the anchor span nor moments from either span can be transmitted across the connection.
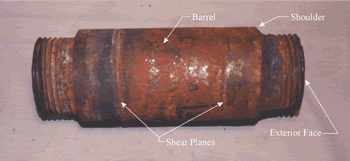
This corrosion can have two detrimental effects on the pin. First, the cross-section of the pin can decrease due to corrosive section loss. This corrosion can produce pitting that may act as crack-initiation sites. Second, corrosion can effectively lock the pin within the connection so that no rotation about the pin is permitted. This can lead to large torsional stresses within a reduced section of the pin. The torsional stresses, combined with the shear stresses, provide a likely location for the development and propagation of cracks and the eventual failure of the pin.
Locating cracks that start on the pin barrel at the shear plane perimeter is a difficult task. The shear plane is not visible unless the pin is removed from the connection - a labor- and equipment-intensive task. Ultrasonic field inspection provides the requisite combination of simplicity of inspection procedure and accuracy of results to make it a preferred method for many bridge owners. Furthermore, ultrasonic techniques access internal pin regions that otherwise are inaccessible except by more costly nondestructive techniques.
Bridge Description
The bridge studied for this research is a 12-span, 324.5-meter- (1064-foot-) long structure that provides access to a heavy-freight transfer facility in the midwestern United States. The bridge deck accommodates two lanes of traffic and is supported by a superstructure consisting of four welded steel plate girders. The bridge contains 12 pin-and-hanger connections, all of which occur at expansion joints approximately 1.5 meters (5 feet) from an adjacent pier. The hanger pins in the bridge all have a barrel diameter of 76 millimeters (3 inches) and an overall length of 216 mm (8.5 in). The distance from the exterior face of the pin to the pin shoulder is 32 mm (1.25 in), and the distance from each exterior face to the closer shear plane is 76 mm (3 in). The bridge is owned and maintained by a state department of transportation (DOT).
The bridge serves as the primary roadway access point to a freight transfer facility and, as such, is subjected to extremely heavy and frequent truck traffic. Permit loads routinely use this structure. A structural analysis of the bridge conducted in 1994 revealed that the pin-and-hanger detail was being subjected to higher stresses than originally intended. The bridge was last inspected in 1996, at which time a National Bridge Inspection Standards (NBIS) routine inspection was conducted. In conjunction with this routine inspection, the DOT contracted a consulting engineer to perform a special inspection of the pin-and-hanger detail; the inspection included detailed measurement of member sizes and expansion-joint gaps. The purpose of these measurements was to determine if the pin-and-hanger detail was "frozen" or locked. In addition, in 1994, a field ultrasonic inspection of the pin members was carried out. The combined results of the ultrasonic testing and visual examination revealed shallow defects in some of the pins and no defects in the hanger links. In general, these shallow defects were reported as wear grooves and/or corrosion section loss having a depth ranging from minimal to 3 mm (one-eighth inch).
In spring 1998, a DOT employee noted that one of the hangers was no longer aligned correctly with its lower pin. An immediate close-up inspection was performed and confirmed that the pin had fractured. As a result, both pins were removed from that connection and were replaced with new pins.
In summer 1998, NDEVC staff members had the opportunity to view the salvaged pin, which had been removed by flame-cutting. The flame-cutting had obscured most of the failed surface; however, enough was visible to conclude that fatigue had played a part in the failure mechanism. The state DOT agreed to permit the NDEVC staff to access to the remaining pins to investigate whether or not additional pins within the structure were cracked.
Field Ultrasonic Inspection
With the state's permission, the FHWA NDEVC conducted ultrasonic testing of the remaining 22 pins on the bridge. The inspection team was led by an American Society of Nondestructive Testing- (ASNT-) certified Level III ultrasonic inspector. The ultrasonic testing was conducted with the intent of identifying cracked or failed pins. The inspections were conducted from a boom lift positioned below the structure. Prior to ultrasonic testing, the pin exterior faces were prepared by grinding to remove paint and smooth the surface to facilitate sound transmission into the pin. The procedures for ultrasonic testing outlined in the Bridge Welding Code were followed throughout this inspection.2
Ultrasonic examinations were performed using a Krautkramer/Branson USN 52 model ultrasonic flaw detector. This instrument features state-of-the-art digital electronics, an electro-luminescent display, and 70 data set storage registers. Preliminary scans were performed using a straight-beam (0 degrees) transducer, with subsequent scans using an incident angle of 15 degrees. An incident angle of 15 degrees was selected to avoid the pin shoulder and to provide a better angle of incidence to the near-side shear plane. A transducer frequency of 5 MHz and a 12.7-mm (0.5-in) element size were used throughout the testing. Prior to testing, the transducer and flaw detector were calibrated to establish sensitivity and time base. Standard American Welding Society (AWS) calibration blocks and specially prepared pin calibration standards, developed by the ultrasonic inspector, were used.
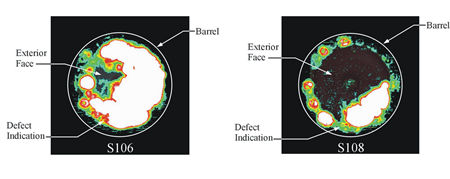
A straight-beam scan was initially used to confirm pin geometry. Measurements of component length were made using a tape measure to corroborate the ultrasonic findings. The straight-beam scan was also used to identify large cracks or complete failure. The angled-beam scans were then conducted to more accurately resolve reflectors located near the pin barrel surface and to examine areas shielded from the straight-beam scan, such as the pin shoulder region. Angled-beam scans were concentrated on pin shear planes where the potential for wear grooves, corrosion, and cracking indicators is greatest. All scanning proceeded in a systematic manner to ensure complete coverage of the pin interior. Scanning included movement of the transducer over the entire pin end with the angle oriented toward the pin barrel. All pins were examined from both exterior faces. In several instances, the nut was not fully seated on the pin end, thereby limiting transducer positioning near the perimeter of the pin end. After testing, all pin ends were painted using a rust-inhibiting primer.
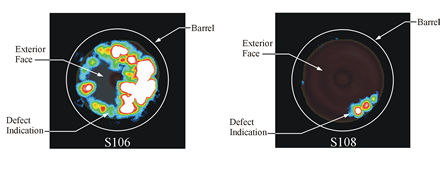
The field inspection indicated that two of the 22 original pins were cracked. This inspection also found five additional pins that had wear-groove indications at the shear plane. All seven of these pins were removed from the bridge structure and were shipped to NDEVC. These pins, labeled S104 through S110, are now part of NDEVC's Component Specimen Database.
This study primarily focuses on the two cracked pins. The results from field ultrasonic testing of the two cracked pins, labeled S106 and S108, are shown in figure 4. The approximate defect profile at the shear plane is indicated in the figure for each pin. The original sketches were produced in the field by hand-sketching.
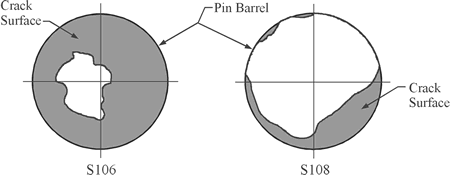
Immersion Tank Ultrasonic Testing
Immersion tank ultrasonic testing of the seven hanger pins was performed at NDEVC. The purpose of this testing was to accurately detect and quantify crack-like defects that may be present in any or all of the pins.
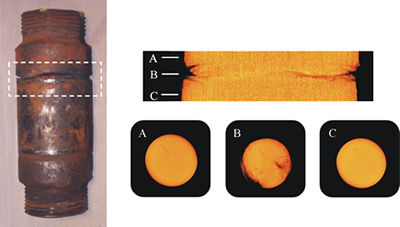
The immersion tank ultrasonic examinations were performed using a system developed by Scanning Systems International Inc. (formerly Infometrics Inc.). TestProÔ, release 6.0, was the software used. The basic setup of the scans can be seen in figure 5. The pin was placed on end while immersed in a water-filled tank. The ultrasonic transducer was positioned above the pin with its face parallel to the end face of the pin. A 5-MHz, 12.7-mm (0.5-in) unfocused transducer was used, with a 60-mm (2.35-in) separation between the transducer face and the pin face. The movement of the transducer for the scanning process was computer-controlled, allowing the electric motors to systematically move the transducer within the plane parallel to the exterior face of the pin. This equipment setup was used for all scans performed in this study.
The system was calibrated with a standard distance-area amplitude block. This block consists of a 51-mm- (2-in-) diameter stainless steel cylinder that is 89 mm (3.5 in) tall. A 3.2-mm- (0.125-in-) diameter flat-bottomed hole (FBH) that is 12.7 mm (0.5 in) deep was present in the center of one end of the reference block. This reference block was chosen since it provides a pulse path from transducer to defect that is similar to that used in hanger pin specimens. This reference block was used to determine the beam spread and the required threshold levels for various transducer gain and attenuation configurations. As a result, a defect location and the extent on a particular plane within the pin (as related to the transducer location) could be determined.
Initial scans were performed on all seven pins. The pins were scanned from both exterior faces to provide full coverage within the pin barrel. This setup allowed for the detection of virtually all reflectors, including most defects shrouded from direct detection by the shoulder of the pin. The identification of defects in the shrouded area through the use of beam spread is critical because this portion of the pin is not directly visible to the straight-beam ultrasonic transducer.
These initial scans indicated that only pins S106 and S108 contained cracks and that the cracks in these pins occurred at the level of the shear plane. Detailed scans were then performed on these two specimens to precisely locate the defects. This additional testing was conducted in two phases. First, the testing apparatus was set up to generally locate small defects near the perimeter of the pin body, below the shoulder. Second, the testing apparatus was set up to precisely locate any defect that occurred within the body of the pin directly under the exterior face.
The specimen preparation and test setup for the detailed phases of testing were as follows. First, the end of each pin that was closer to the defect was milled flat and perpendicular to the body of the pin. This was done to optimize transmission of the ultrasonic pulses into the pin. In addition, because the approximate location of the defects had been determined by the previous scans to be at the level of the shear plane, these further scans were optimized for a 19-mm- (0.75-in-) deep zone at the level of the shear plane. Finally, these scans were set to provide a much finer spatial resolution (0.25 mm by 0.25 mm).
As mentioned previously, the first phase of the detailed testing focused on the identification of the location of defects near the perimeter of the body of the pin. These scans used the same gain, attenuation, and threshold settings as were used for the initial preliminary scans of all seven pins. Through the use of a calibration block, it was determined that these settings allowed for the scan to receive a reflection from a defect at the level of the shear plane, which was 6.4 mm (0.25 in) outside of the direct path of the transducer. Figure 6 shows the beam spread of the ultrasonic pulse that allows for these defects to be located. Accordingly, the procedure could detect a defect that was near the perimeter of the pin body at the level of the shear plane. However, it must be noted that crack-like indications at the limits of this range were difficult to differentiate from wear grooves or corrosion.
The results of these scans are presented in the form of a Cscan image in figure 7. (Within ultrasonics, there are different ways of looking at the ultrasonic data, and these are called Ascans, Bscans, and Cscans.) For reference, the Cscan image of the defects has been superimposed over a darkened Cscan image of the exterior face of the pin. This allows for clear identification of the travel path of the ultrasonic pulse. The outline of the pin barrel is also presented.
The second phase of the detailed investigation of the cracked shear plane regions was then conducted. The goal of this set of scans was to accurately locate the defects that lay directly under the exterior face of the pin. The calibration block was used to determine what settings were required so that only defects that were at least partially under the transducer were reported. The Cscan results for these tests are shown in figure 8. Once again, for reference, the exterior face of the pin and the pin barrel are presented.
Using the results shown in figures 7 and 8, a schematic representation of the defects present at the shear plane level of S106 and S108 can be determined. These results are shown in figure 9. The cracked portion of the shear plane is shaded in the figure.
Comparison of Field and Immersion Tank Ultrasonic Results
Comparison between the field ultrasonic results (figure 4) and the immersion tank ultrasonic results (figure 9) indicates that the two methods provide similar conclusions as to the extent of cracking at the shear plane level in these two specimens. In pin S106, the immersion tank findings indicate that 79 percent of the cross-section is cracked, and the field ultrasonics indicate that 83 percent of the section is cracked. For S108, the immersion tank and field ultrasonic findings indicate, respectively, that 30 and 22 percent of the section is cracked. Due to the automated nature and the higher spatial resolution of the immersion tank scan, the results from this portion of the testing are believed to provide a more refined and more accurate representation of the extent of the cracking. However, the results from the field ultrasonic testing were found to be quite accurate, given the logistical challenges present in the acquisition of field ultrasonic data.
Computed Tomography
Computed tomography (CT) was also used to inspect pin S106. The CT data were acquired with a 9-MV LINAC x-ray source and an area-array digital detector. Figure 10 shows a small portion of the CT results. The images produced by the CT scans clearly show that a significant crack exists at the level of the shear plane in this specimen. At this time, a full reconstruction of the data has not been performed, and therefore, a quantitative assessment of the size of the crack is not possible. However, the location, general shape, and general size of the crack correspond well with the results obtained from the ultrasonic testing.
Conclusions
Field and immersion tank ultrasonic inspection techniques were used to investigate possible defects within hanger pins removed from an in-service bridge. The field inspections identified crack-like defects within two pins. These pins were sent to the FHWA's NDEVC for further testing. The results from the immersion tank testing correlated well with the field ultrasonic testing. Both the defect location and defect size findings show a high level of consistency between the two ultrasonic techniques.
References
1. R.A. Walther and R.D. Gessel. "Ultrasonic Inspection of Bridge Pin and Hanger Assemblies," Proceedings, Structural Materials Technology, An NDT Conference, 1996, San Diego, Calif., p. 23-28.
2. Bridge Welding Code, ANSI/AASHTO/AWS D1.5-98, American Association of State Highway and Transportation Officials, Washington, D.C., 1998.
Benjamin A. Graybeal is a research engineer employed by Wiss, Janney, Elstner Associates Inc and working at the Federal Highway Administration's Nondestructive Evaluation Validation Center. He received his master's degree in structural engineering from Lehigh University and is currently enrolled in the doctoral program in structural engineering at the University of Maryland.
R.A. Walther is a consultant for Wiss, Janney, Elstner Associates Inc.
Glenn A. Washer is the program manager of the Federal Highway Administration's Nondestructive Evaluation Validation Center at the Turner-Fairbank Highway Research Center in McLean, Va. He has a master's degree in civil engineering from the University of Maryland. He is currently a doctoral candidate at The Johns Hopkins University Center for Nondestructive Evaluation. Washer is a licensed professional engineer in Virginia.
Amy M. Waters of the Lawrence Livermore National Laboratory was instrumental in the performing of the computed tomography work.